
The need for sustainable engineering practices has become demanding as the global community works toward achieving the United Nations’ Sustainable Development Goals (SDGs). This has increased the emphasis on environmentally friendly engineering solutions that utilise natural processes.
Most civil engineering infrastructure (i.e., buildings, roads, dams) are often constructed on the soil or rocks (known as geomaterials) that make up the earth’s subsurface. These geomaterials, while essential, sometimes fall short in their natural state, lacking the strength and permeability needed for robust construction. To address this problem, ground stabilisation methods are employed to improve the engineering behaviour and meet design standards. Though effective, traditional ground stabilisation methods can be resource-intensive and environmentally harmful, prompting the need for more sustainable and eco-friendly alternatives.
One such pioneering alternative method is biocementation, a process that utilises microbial activity to improve the properties of geomaterials. Microorganisms are plentiful on the ground we walk on. Certain microorganisms, particularly bacteria, can naturally interact with soil minerals and induce the precipitation of calcium carbonate – a natural cementing agent. By leveraging these microbial processes, we can sustainably improve soil strength and reduce permeability. Microbially Induced Calcite Precipitation (MICP) is one of the most widely used biocementation techniques, which utilises the bacteria Sporosarcina pasteurii to hydrolyse urea, leading to the precipitation of calcium carbonate (biocement). The precipitated biocement fills the pores between soil particles and improves engineering behaviour. Consequently, the MICP has several applications in ground stabilisation, plugging leaks in carbon geo-sequestrated aquifers, subsurface barriers, and mitigating erosion.
In the MICP treatment, the bacterial concentration is the major element that releases enzymes by hydrolysing urea, thereby contributing to significant precipitation (CaCO3). Therefore, the amount of biomass plays a substantial role in the effectiveness of the process, but it is influenced by phenomena such as biomass encapsulation and bacterial attachment. Typically, bacterial encapsulation initiates during precipitation, as precipitated biocement covers the attached biomass surface, leading to the inactivation of bacteria for the reaction with chemicals in the pore fluid, thus limiting the urea hydrolysis rate. The attachment of bacteria is another significant phenomenon, which transfers the mass of bacteria from a suspended state in water to an attached state onto the solid grains, which is crucial for biocementation. Without attachment, the bacterium could flush out of the system, resulting in lower calcite precipitation rates. Therefore, a fundamental understanding of biomass encapsulation and attachment is essential for ensuring the efficiency of MICP treatments, even at field scales.
In order to fundamentally understand the MICP treatment and its related mechanisms, knowledge of biology (B), chemistry (C), hydraulics (H), and mechanics (M) is essential. Therefore, considering the complexity of the problem and difficulty in understanding interactions at a laboratory scale, the numerical models are valuable for predicting and optimising the process with relevant physics (i.e., bio-chemo-hydro-mechanics, BCHM).
In a previous study, the authors, Mr. Pavan Kumar Bhukya, Ms. Nandini Adla, and Prof. Dali Naidu Arnepalli from the Department of Civil Engineering, Indian Institute of Technology (IIT) Madras, Chennai, India, had come up with a numerical model to evaluate the rate of bacterial encapsulation, its effect on the precipitated biocement content and permeability. The authors proved that considering the bacterial encapsulation effect on urea hydrolysis rate was necessary and influenced the amount of calcite (calcium carbonate) precipitation.
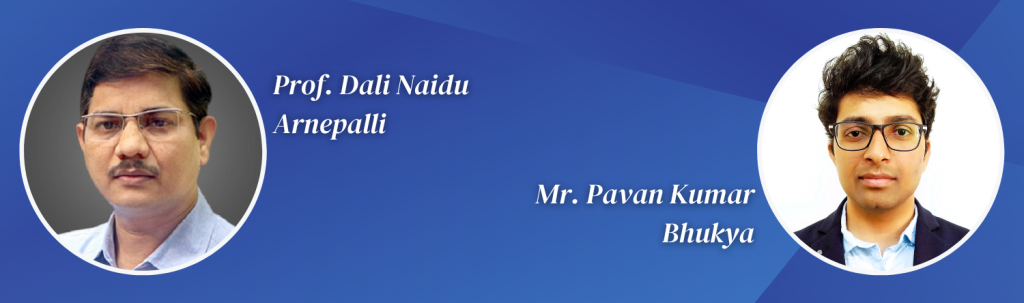
In the present study, the authors, Mr. Pavan Kumar Bhukya and Prof. Dali Naidu Arnepalli, have focussed on the role of bacterial attachment, developing a BCHM model that takes into account simple and sophisticated attachment rate models which include constant, exponential, gamma, and colloidal models.
The study examined the influence of different bacterial attachment models on biomass distribution and the resulting biocement content. While most research tends to focus on accurately capturing calcite distribution, the bacterial profile plays a crucial role in determining the correctness of these predictions. If bacterial concentrations are misrepresented, the resulting calcite distribution may not reflect the actual physical process. Therefore, it is essential first to calibrate (measure) bacterial distribution to ensure that the attachment rate accurately represents the actual attachment process. Without proper calibration, inaccurate predictions of biomass attachment could have significant consequences, particularly in large-scale applications, if not for laboratory-scale investigations.
With this as a prime goal, the present study developed a numerical model to evaluate the influence of the bacterial attachment models on the BCHM behaviour during the biocementation process. A workflow was also proposed to calibrate (measure) the parameters of all the attachment rate models from suspended bacterial profiles. It was found that the gamma and colloidal attachment theories reasonably predicted the experimental response of suspended biomass distribution and calcite content for the considered test case. However, constant and exponential attachment models overpredicted the calcium carbonate content. All the attachment rate models predicted the permeability changes accurately.
The study underscores the potential applications, future developments of the BCHM model, and adoption of an attachment model to address geotechnical and geoenvironmental engineering problems. This study also reveals that using a constant attachment model for all scenarios will not suffice for reasonably capturing biomass distribution and calcite content. The research highlighted that choosing the suitable bacterial attachment model is critical for accurate predictions in soil bio-cementation. Incorrect model choices can lead to significant errors in estimating calcite content and soil strength. The study also highlights the importance of considering various factors, such as injection strategy, bacterial concentration, and soil properties, to refine attachment models and enhance their predictability. In the future, the authors feel that the work should be extended for several field-scale injection strategies to better predict attached biomass concentration and calcite content. Overall, the study highlights the importance of selecting the correct attachment model to enhance predictability and effectiveness in soil biocementation.
Dr. David Landa Marban, a researcher from the Energy and Technology Division, NORCE Norwegian Research Centre, Bergen, Norway, acknowledged the importance of the work done by the authors with the following comments: “Microbially induced calcite precipitation (MICP) is a bio-geochemical process with promising applications, such as sustainable geomaterial stabilisation. The effectiveness of MICP is fundamentally governed by bacterial attachment, as the bacteria on soil grains significantly contribute to enzyme concentration. These enzymes catalyse the biochemical reaction of urea hydrolysis, resulting in calcium carbonate precipitation, also known as biocement. The study by Prof. Dali Naidu Arnepalli and his Ph.D. student Pavan Kumar Bhukya presents a comprehensive MICP mathematical model, focusing on the bacterial attachment process. By evaluating various bacterial attachment models and their impact on calcite precipitation, the study underscores the importance of selecting an appropriate model to accurately predict attached biomass concentration and biocement content. The results quantify the influence of these models on bacterial and calcium carbonate profiles by comparing them with experimental data. Additionally, the study recommends specific models for different subsurface conditions. In summary, this research provides valuable insights into the bacterial attachment mechanisms that initiate biocementation within soil pore spaces.”
Article by Akshay Anantharaman
Here is the Tech Talk article link to the authors’ previous work
Click here for the original link to the paper